Low pressure just off the coast of South Carolina (0900 UTC map analysis) brought wide-spread fog and low ceilings to the southeastern United States on 7 February. The toggle below shows the Night Fog Brightness Temperature Difference (BTD, 10.3 µm – 3.9 µm) field that is often used to highlight regions where clouds made up of water droplets are widespread. The night time microphysics RGB includes as its green component the Night Fog BTD, and the correlation between the blue/cyan enhancement in the BTD and the cyan/yellowish color in the RGB is obvious. A challenge in using those two fields for low fog/stratus detection arises where multiple cloud layers might exist such that the BTD is not highlighting low cloud — because mid-level or high clouds block the satellite view of low clouds. This shortcoming is mitigated in IFR Probability fields by including information (from the Rapid Refresh model) on low-level saturation. Thus, IFR Probability fields suggest a greater likelihood for low clouds over coastal South Carolina (for example). If low-level saturation is *not* indicated in the model, IFR Probabilities will show minimal values, as over central Georgia (near Atlanta, especially), for example.
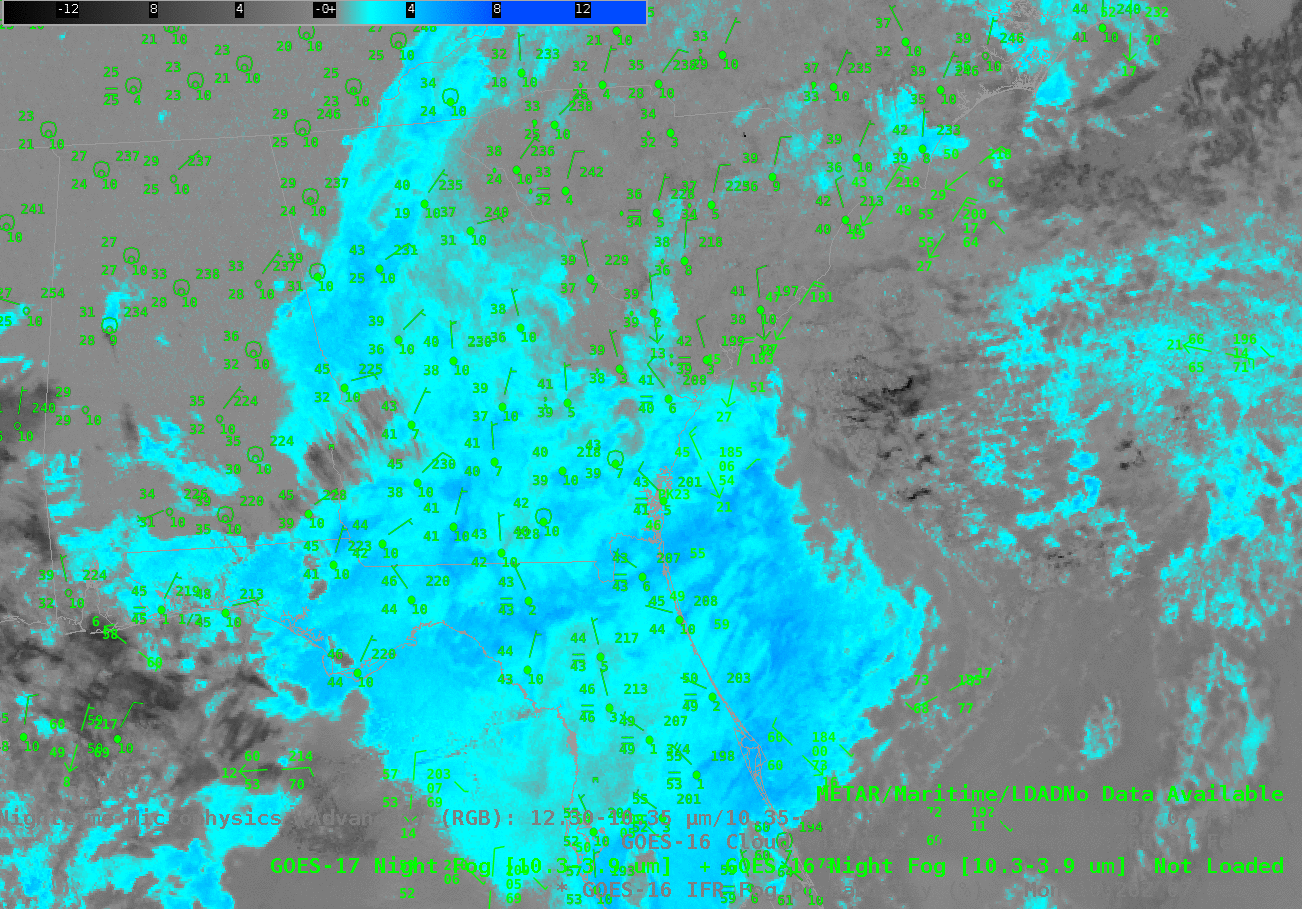
At 1156 UTC, a similar distribution to the three fields continues. Note how the presence of cirriform clouds over south-central Georgia affects the fields. The Night Fog BTD and Night Microphysics RGB both change radically, and IFR probabilities reduce — in part because the algorithm is less confident that low clouds exist. The small IFR Probabilities continue around Atlanta’s airline hub, important information from an aviation standpoint!
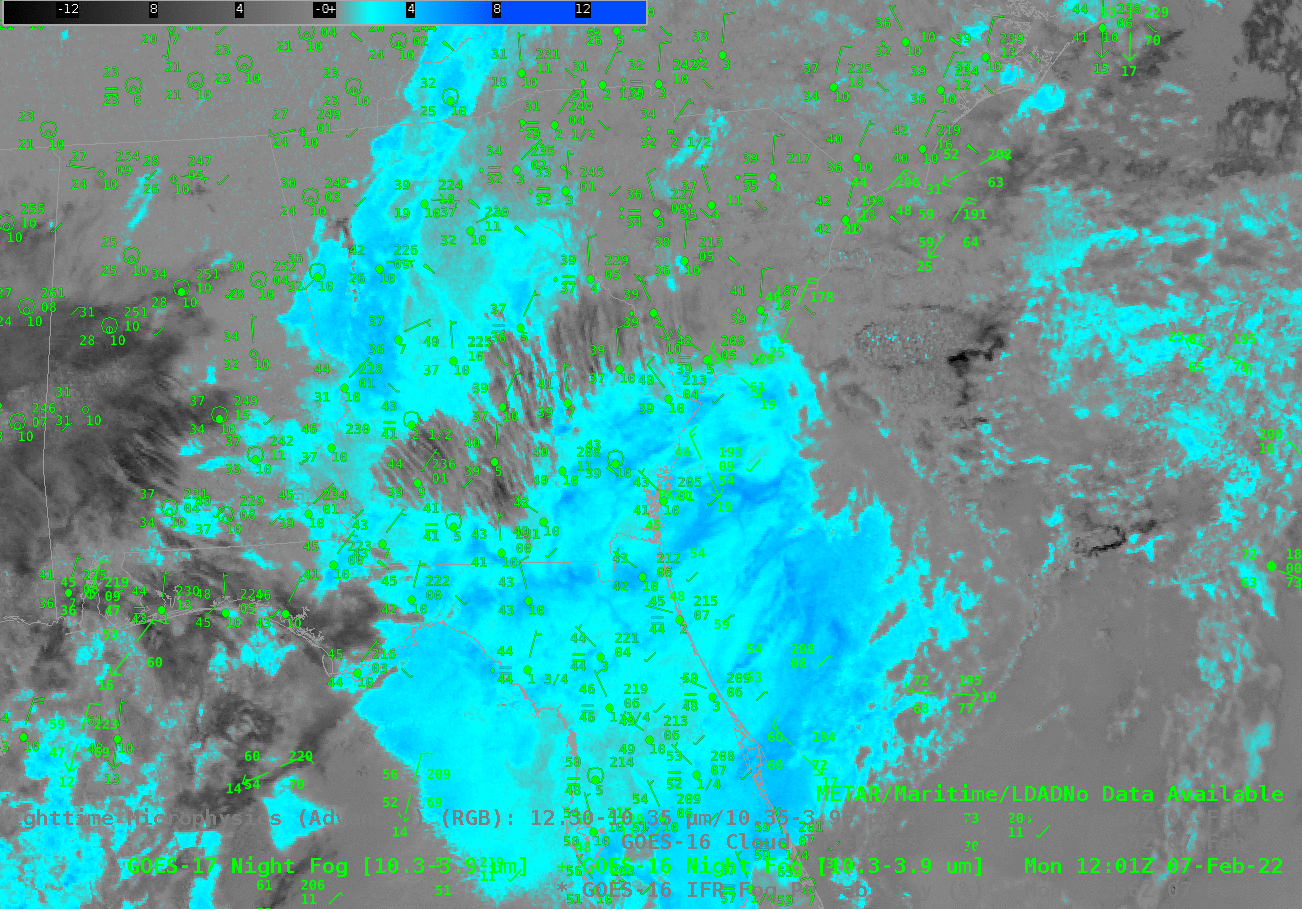
The toggle below compares IFR Probability with GOES-R Cloud Thickness. This is close to the time around sunrise/sunset when GOES-R Cloud Thickness are not computed because of quickly changing reflected solar shortwave infrared (3.9 µm) radiation; indeed, the cutoff can be viewed in the SSW-NNE terminator line over the Atlantic at the eastern edge of this image. If the low clouds on this day were strictly radiation (a dubious claim in the presence of rain!), then this scatterplot could be used to help decide when conditions might clear.
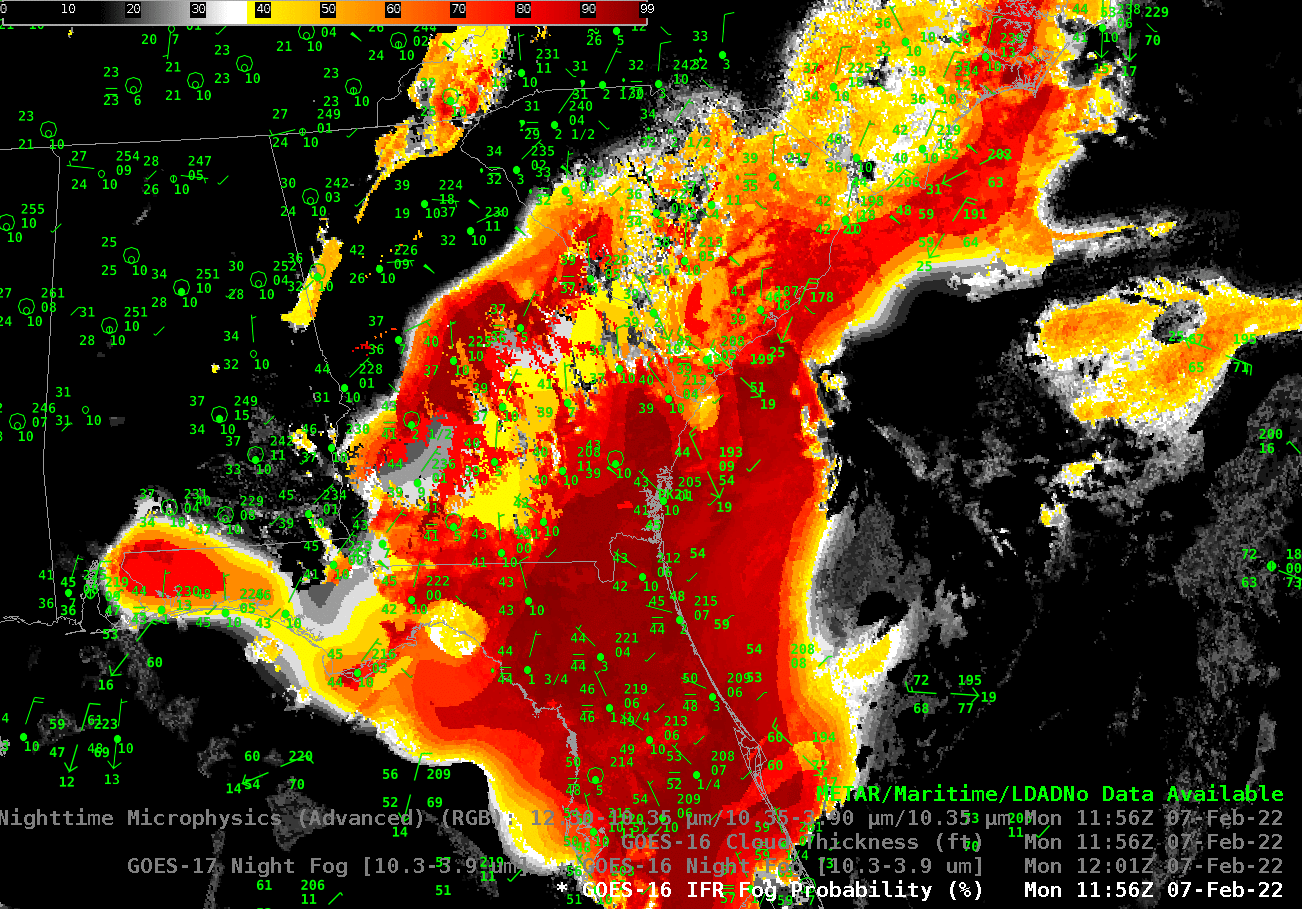