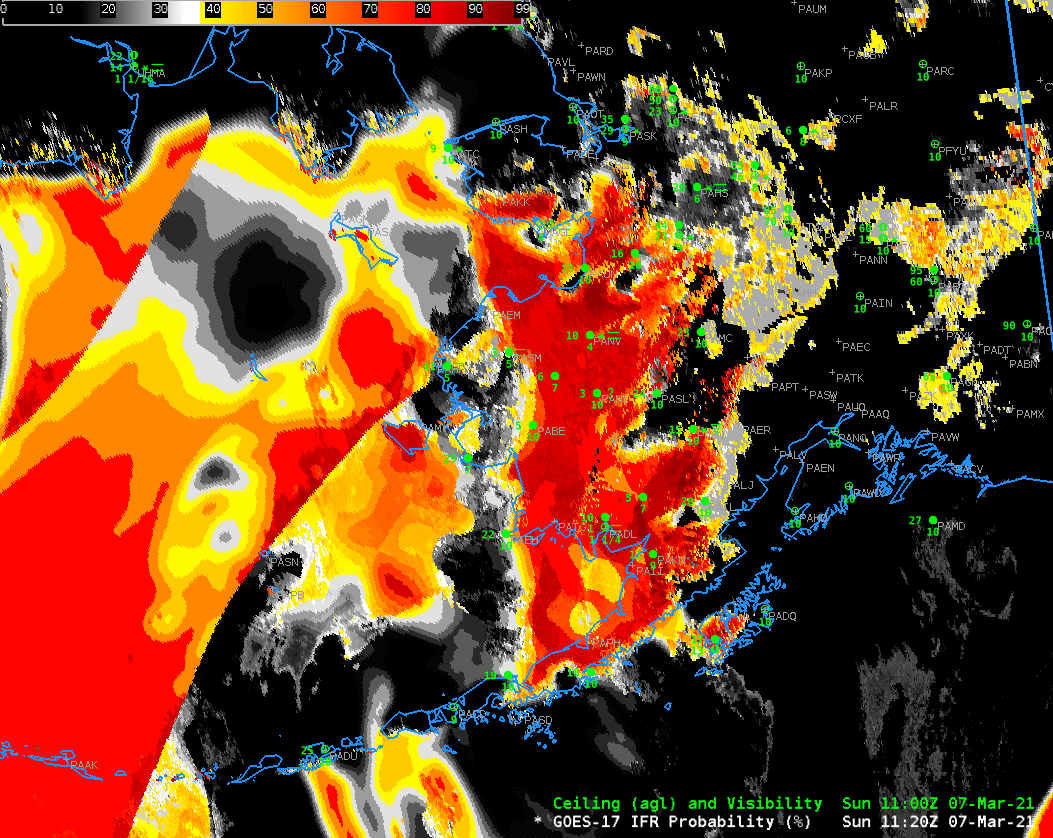
GOES-17 IFR Probability fields over southwestern Alaska and the Aleutians, shown above, characterize several areas of likely IFR conditions on 7 March. One region is over the Bering Sea, north of the Aleutians. This is a region where multiple cloud layers prevent the satellite from viewing low clouds. However, the Rapid Refresh model simulation there does show saturation at low levels; large values of IFR Probability are the result. When model fields control the IFR Probability values, there is little pixel-to-pixel variability and field has a uniform look. This example also shows values from two different models — the Alaska version of the Rapid Refresh (over most of the domain) and the GFS model over parts of Asia.
A second region of high IFR Probabilities is over land in southwest Alaska. There, an absence of high clouds means that satellite detection of low clouds occurs. There is good agreement between IFR Probability fields and observations of ceilings and visibility. For example, note how IFR Probability decreases around PAUN (Unalakeet) as the visibility improves and ceilings lift.
The toggle between the Night Fog Brightness temperature difference and IFR Probability field at 1210 UTC, below, reveals differences as well. The Brightness Temperature Difference field shows uniformity (except for a path of high clouds centered on Dillingham). IFR Probability fields show that the low clouds and reduced visibilities are not so widespread: note the conditions near McGrath and Sleetmute — IFR Probabilities are low, and IFR conditions are not present.
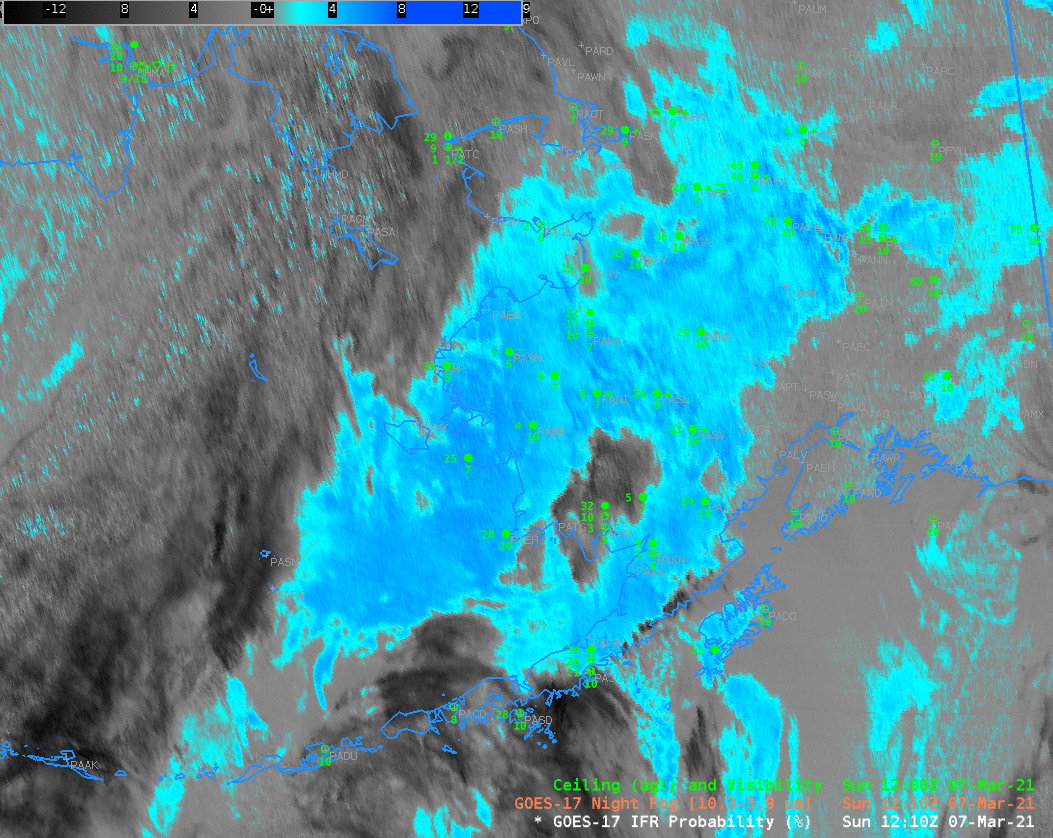
The animation of Night Fog Brightness temperature below demonstrates challenges in using the field. When high clouds overspread a region, the low cloud signal is lost; perhaps because the fog lifts, and perhaps not. The signal is also lost as the sun rises: the emissivity differences that drive the difference field at night are overwhelmed by solar reflectance as the sun rises.
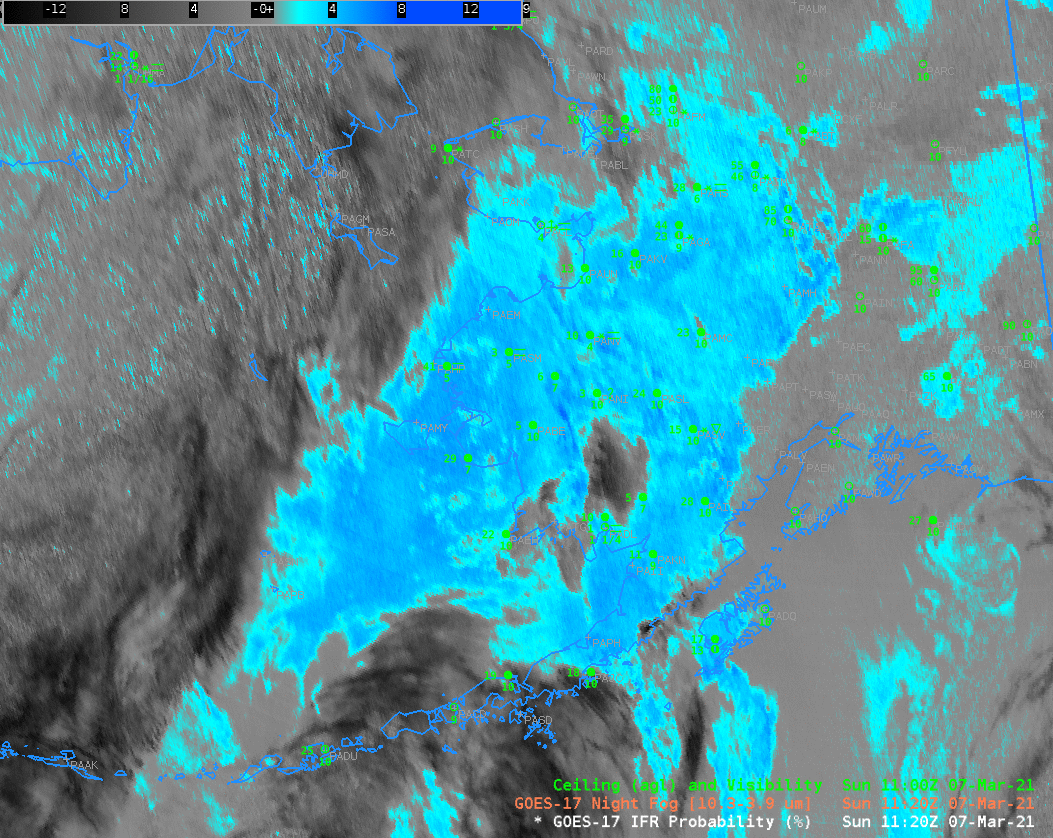