Ice Fog at Denver International Airport on Sunday 30 November resulted in the diversion of almost 50 flights. (News Link) From the link:
Sunday morning fog caused about 46 flights scheduled to land at Denver International Airport to be diverted, airport officials said.

GOES-R IFR Probability Fields, every 15 minutes, from 0500 UTC through 2200 UTC on 30 November 2014, along with surface reports of ceilings and visibility (Click to enlarge)
The GOES-R IFR Probability field gave useful anticipatory information for this event. The animation above shows a line of high IFR Probability moving southward and westward. Stations within the highest IFR Probability reported freezing fog (e.g. Sidney Nebraska (KSNY) at 0800 UTC, Akron, CO (KAKO) at 1200, 1300 and 1400 UTC and Kit Carson Airport in Burlington CO (KITR) at 1400 UTC). When the region of higher IFR Probability abuts up against Denver International (KDEN), then, at 1600 UTC, the Freezing Fog that occurred should not surprise. This region of enhanced IFR Probability persisted near Denver International through 2200 UTC.
The METARS, listed below, show the onset of the freezing fog (FZFG). Note that times are boldface in black, and fog-related observations are boldfaced in red:
KDEN 301353Z 13005KT 10SM FEW110 BKN220 07/M13 A2981 RMK AO2
SLP068 T00721128 $=
KDEN 301453Z 17007KT 10SM FEW110 SCT220 06/M11 A2984 RMK AO2
SLP082 FG BANK DSNT NW-SE T00561111 51034 $=
KDEN 301539Z 07016KT 1/2SM R35L/P6000FT FZFG FEW001 FEW110
SCT220 M06/M08 A2988 RMK AO2 WSHFT 1505 FG FEW001
T10611078 $=
KDEN 301542Z 07017KT 1/4SM R35L/P6000FT FZFG FEW001 FEW110
SCT220 M06/M07 A2989 RMK AO2 WSHFT 1505 FG FEW001 VIS W
1/2 T10611072 $=
KDEN 301553Z 07019KT 1/4SM R35L/2200VP6000FT FZFG VV002
M06/M07 A2991 RMK AO2 WSHFT 1505 PRESRR SLP131 FROPA
I1000 T10611072 $=
KDEN 301630Z 07020KT 1/8SM R35L/1400V2200FT FZFG VV001
M06/M07 A2993=
KDEN 301637Z 08019KT 1/4SM R35L/1400V2400FT FZFG VV001
M06/M07 A2994 RMK AO2 PK WND 07026/1633 TWR VIS 1/4
I1004 T10611072 $=
KDEN 301651Z 08021G28KT 1/4SM R35L/1000V1600FT FZFG VV002
M07/M07 A2995 RMK AO2 PK WND 07028/1643 I1004 $=
KDEN 301653Z 08023KT 1/4SM R35L/1000V1400FT FZFG VV002
M07/M07 A2996 RMK AO2 PK WND 07028/1643 SLP145 I1004
T10671072 $=
(Click here to see a more English Language Listing; Click here to see a meteorogram)
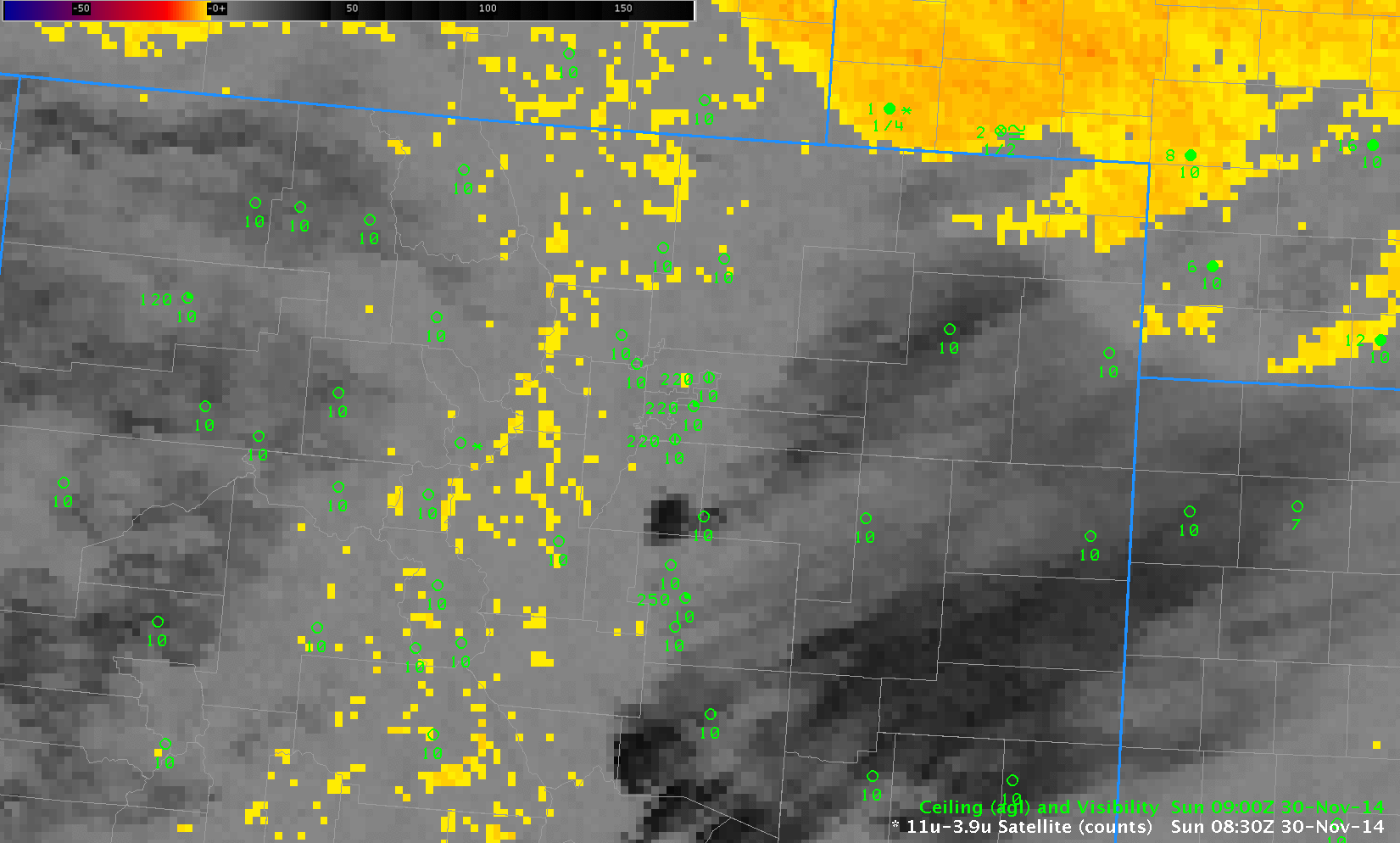
GOES-13 Brightness Temperature Difference fields (10.7µm – 3.9µm) from 0800 through 1600 UTC on 30 November 2014 (Click to enlarge)
For comparison, the Brightness Temperature Difference Field is shown above. Terrain-induced cirrus clouds largely obscured the view of low clouds from satellite in this case. Thus, the incorporation of surface information via the Rapid Refresh model was key to producing an IFR Probability field with useful content.
Visible Imagery from GOES-13 (below) and GOES-15 (bottom) show the cirrus and the underlying low clouds. The steady southward advancement of the low clouds is consistent with the motion of the IFR Probability fields.
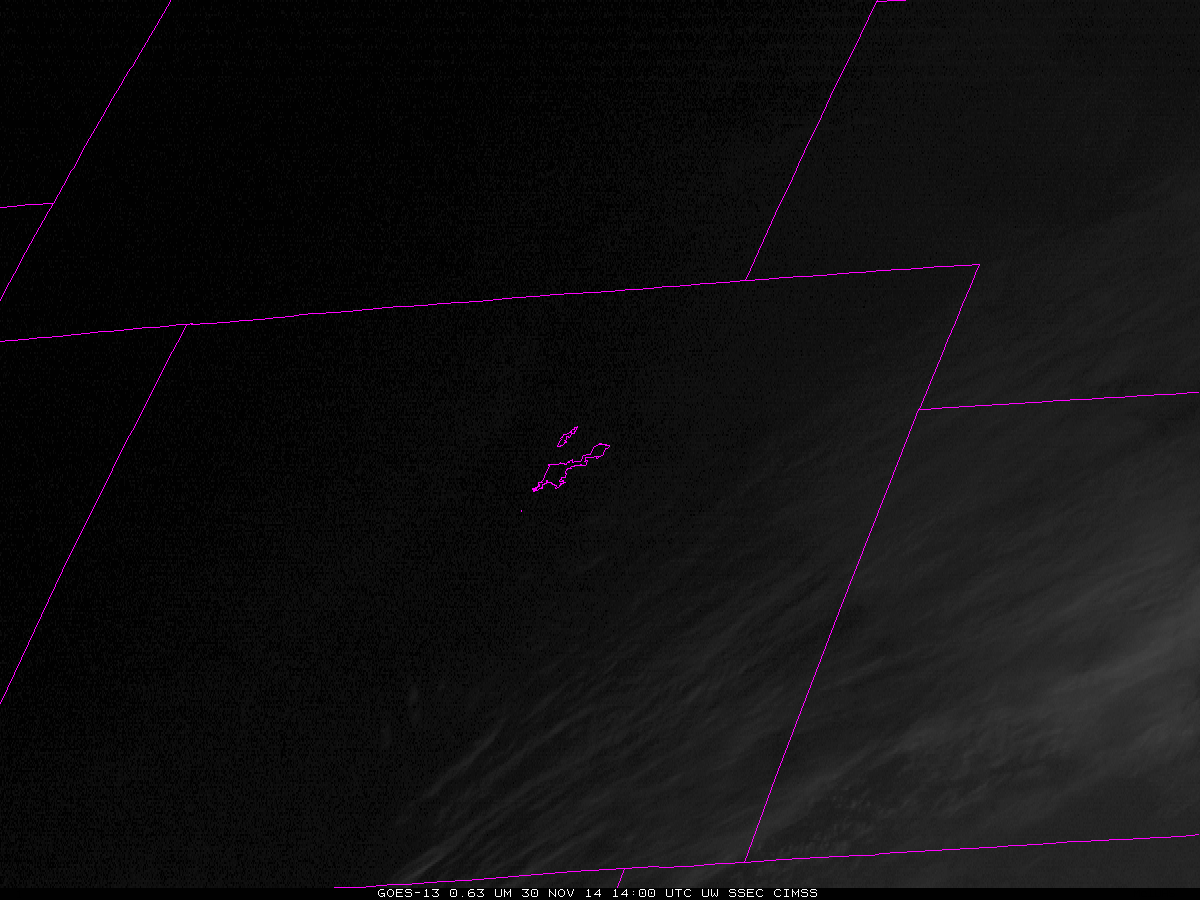
GOES-13 Visible Imagery (0.63µm) animation, 1400-1800 UTC on 30 November 2014 (Click to enlarge)
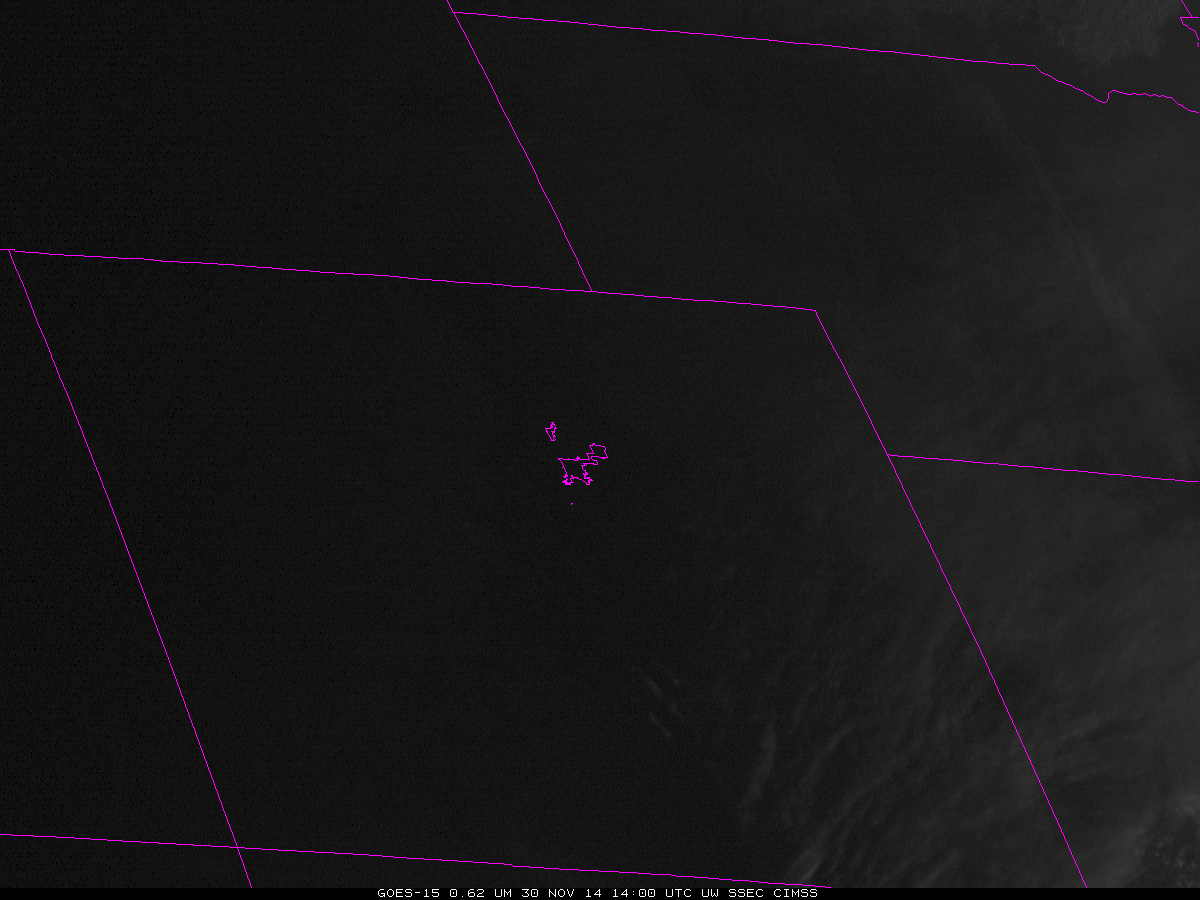
GOES-15 Visible Imagery (0.62µm) animation, 1400-1800 UTC on 30 November 2014 (Click to enlarge)