
GOES-16 Brightness Temperature Difference (10.3 µm – 3.9 µm) at 0912 UTC on 2 October 2017 over the Mid-Atlantic States (click to enlarge)
GOES-16 data posted on this page are preliminary, non-operational and are undergoing testing.
The images above show the GOES-16 Brightness Temperature Difference at the same time at two places over the United States: The mid-Atlantic States (above) and Oregon and surrounding States (below). The ‘Fog’ Product, as this Brightness Temperature Difference is commonly called, in reality identifies only clouds that are made up of water droplets — that is, stratus. A cloud made up of water droplets emits 10.3 µm radiation nearly as a blackbody does. Thus, the computation of Brightness Temperature — which computation assumes a blackbody emission — results is a temperature close to that which might be observed. In contrast, those water droplets do not emit 3.9 µm radiation as a blackbody would. Thus, the amount of radiation detected by the satellite is smaller than would be detected if blackbody emissions were occurring, and the computation of blackbody temperature therefore yields a colder temperature, and the brightness temperature difference field, above, will show clouds made up of water droplets as positive, or cyan in the enhancement above.
The River Valleys of the northeast show a very strong signal that suggests Radiation Fog is developing over the relatively warm waters in the Valleys. The Delaware, Hudson, Mohawk, Connecticut, Susquehanna, Allegheny, Monongahela, and others — all show a signature that one would associate with fog. A signal is also apparent from southern New Jersey southwestward through the Piedmont of North Carolina. Would you expect there to be fog there as well, given the signal?
The State of Oregon at the same time shows a very strong signal in the ‘Fog’ Product. A clue that this might be only stratus, and not visibility-restricting fog, lies in the structure of the clouds — they do not seem to be constrained by topographic features as is common with fog.

GOES-16 Brightness Temperature Difference (10.3 µm – 3.9 µm) at 0912 UTC on 2 October 2017 over Oregon and adjacent States (click to enlarge)
GOES-R IFR Probabilities are computed using Legacy GOES (GOES-13 and GOES-15) and Rapid Refresh model information; Preliminary IFR Probability fields computed with GOES-16 data are available here. These GOES-16 fields should be available via LDM Request when GOES-16 becomes operational as GOES-East.
GOES-R IFR Probability Fields use both the Brightness Temperature Difference field (10.7 µm – 3.9 µm) from heritage GOES instruments and information about low-level saturation from Rapid Refresh Model output. The horizontal resolution on GOES-13 and GOES-15 is coarser than on GOES-16 (4 kilometers at the sub-satellite point vs. 2 kilometers), so small river valleys will not be resolved. (It is also difficult for the Rapid Refresh model to resolve small valleys).
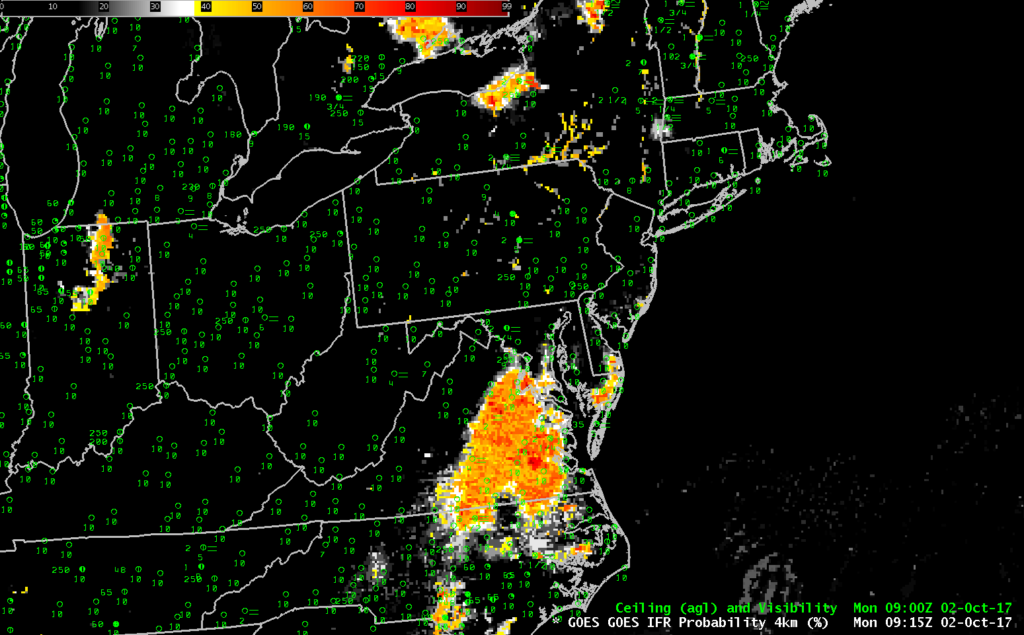
GOES-R IFR Probability fields at 0915 UTC, along with 0900 UTC surface observations of ceilings and visibility (Click to enlarge)
The IFR Probability Fields, above, show some signal over the river valleys of the northeast; that signal is mostly satellite-based, but the poor resolution of GOES-13 means that fog/stratus in the river valleys is not well-resolved. Still, a seasoned forecaster could likely interpret the small signals that are developing to mean fog is in the Valleys. (And restrictions to ceilings and visibilities are certainly reported in the river valleys of the Mid-Atlantic and Northeast) IFR Probabilities are also noticeable over southeast Virginia, although widespread surface observations showing IFR Conditions are not present. (Such observations are somewhat more common near sunrise, at 1130 UTC).
IFR Probabilities are much less widespread over Oregon, with most of the signal over western Oregon related to the topography. In this example, IFR Probabilities are ably screening out regions where elevated stratus is creating a strong signal for the satellite in the Brightness Temperature Difference field.
