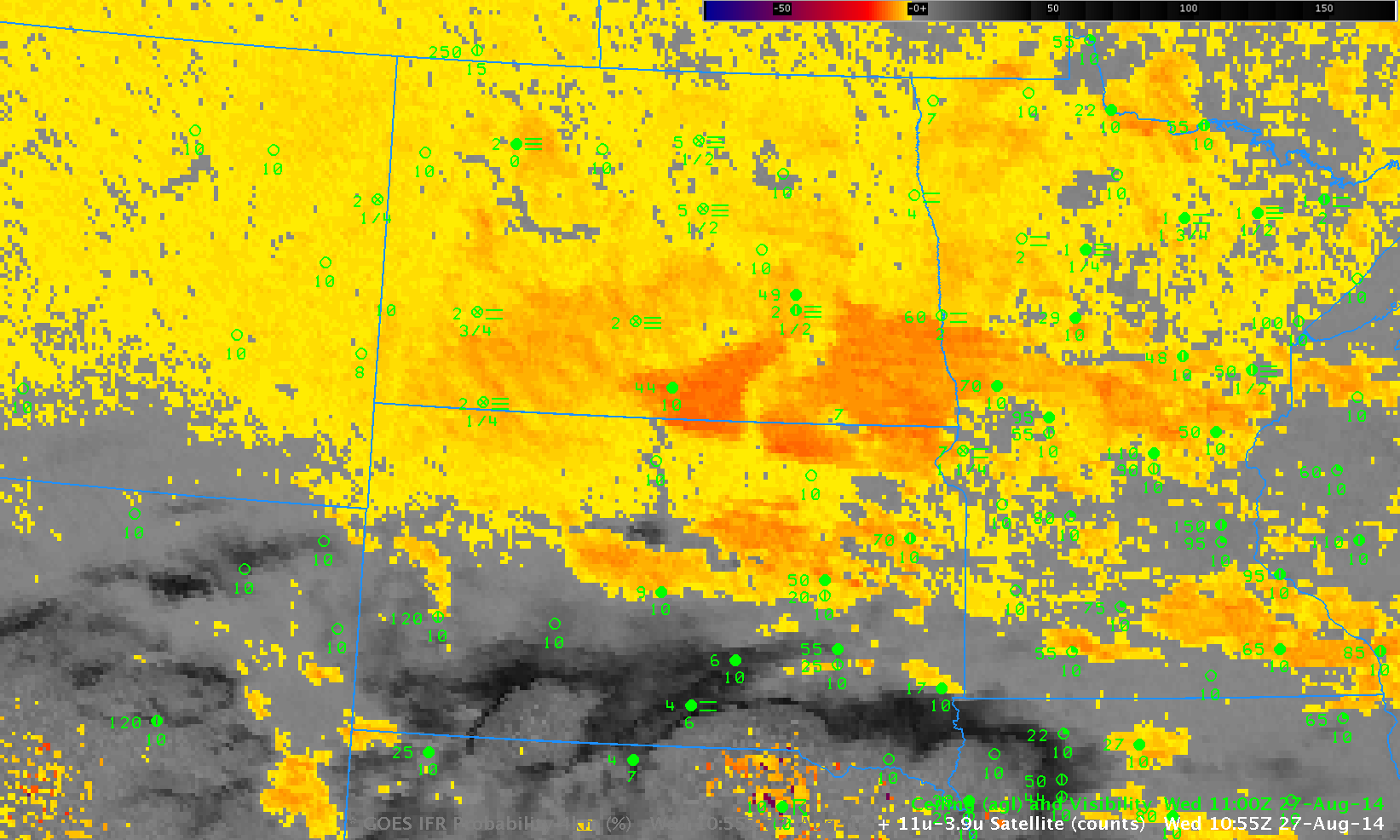
GOES Brightness Temperature Difference (10.7 µm – 3.9 µm) and surface observations of ceilings and visibilities, 1100 UTC, 27 August 2014
GOES data indicated widespread stratus over North Dakota and adjacent states early in the morning on 27 August. The image above demonstrates several difficulties that come with using the brightness temperature difference field to identify regions of reduced ceilings and visibilities. A stratus deck (over eastern Montana) and a fog bank (over central North Dakota) can have a very similar signal in the brightness temperature difference field. Furthermore, regions where ice crystals comprise the clouds (southern South Dakota) show a different signal. Low-level stratus will be invisible to the satellite at night if it is beneath a higher-level cirrus shield.
IFR Probabilities for the same time, below, more accurately define where the lowest visibilities and ceilings occur because Rapid Refresh Model data are used to identify regions where part of the lowest layers of the model atmosphere is saturated. If there is saturation below higher clouds (southern South Dakota), then IFR Probabilities are increased. If there is not low-level saturation in the model in regions where low stratus/fog is present, then IFR Probabilities are reduced (eastern Montana).
Note also how the character of the fields changes if satellite data are not used as an IFR Probability predictor. IFR Probabilities in southern South Dakota (where multiple cloud layers are present associated with a convective feature) have a uniform look to them, as they are derived solely from model-based fields. Over North Dakota and Montana, satellite data can be used as a predictor; the individual satellite pixels are obvious. When only model data are used as predictors, the probability is reduced. Thus, the color yellow in a region of model-only prediction (southern South Dakota) should be interpreted differently than the color yellow in a region that uses both model and satellite predictors (North Dakota and Montana).