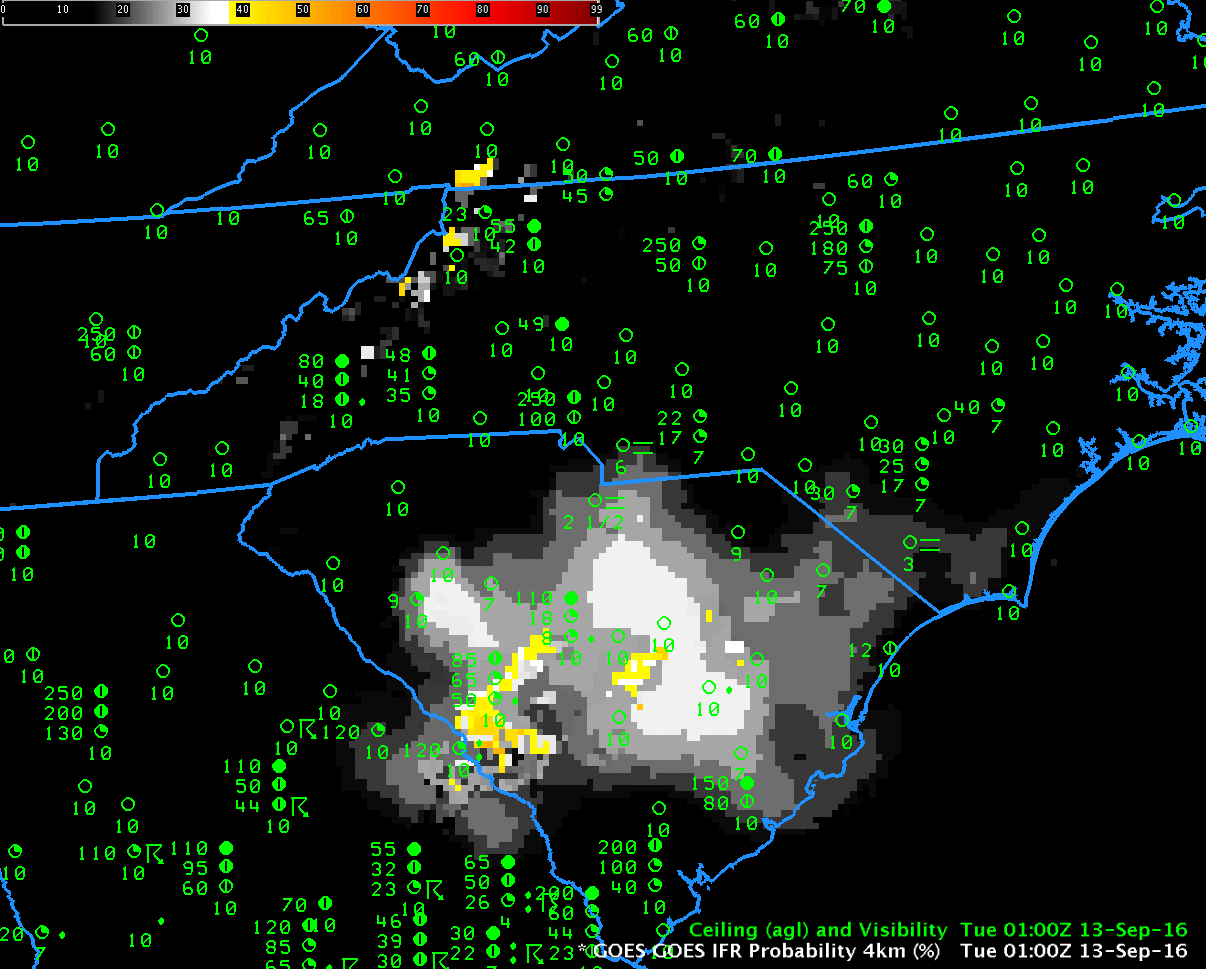
GOES-R IFR Probability Fields and surface reports of ceilings and visibilities, 0100-1000 UTC on 13 September 2016 (Click to enlarge)
High Pressure over the eastern United States allowed Radiation Fog to form over much of the southeast early on the morning of 13 September 2016. The GOES-R IFR Probability hourly animation, above, shows increasing probabilities of IFR conditions over much of North and South Carolina, with IFR conditions observed at many stations by sunrise (graphic from here). IFR Probabilities provided an earlier alert to the fog development (as such, it’s a good situational awareness tool) than was possible from the traditional brightness temperature difference field (see the 0400 UTC image below — click here for a much larger image) because of multiple cloud layers present over the Carolinas in the wake of departing showers. The enhancement for the brightness temperature difference field is such that clouds composed of water droplets are typically shaded orange or yellow. In the 0400 UTC brightness temperature difference field below (right), fog is not indicated over South Carolina.
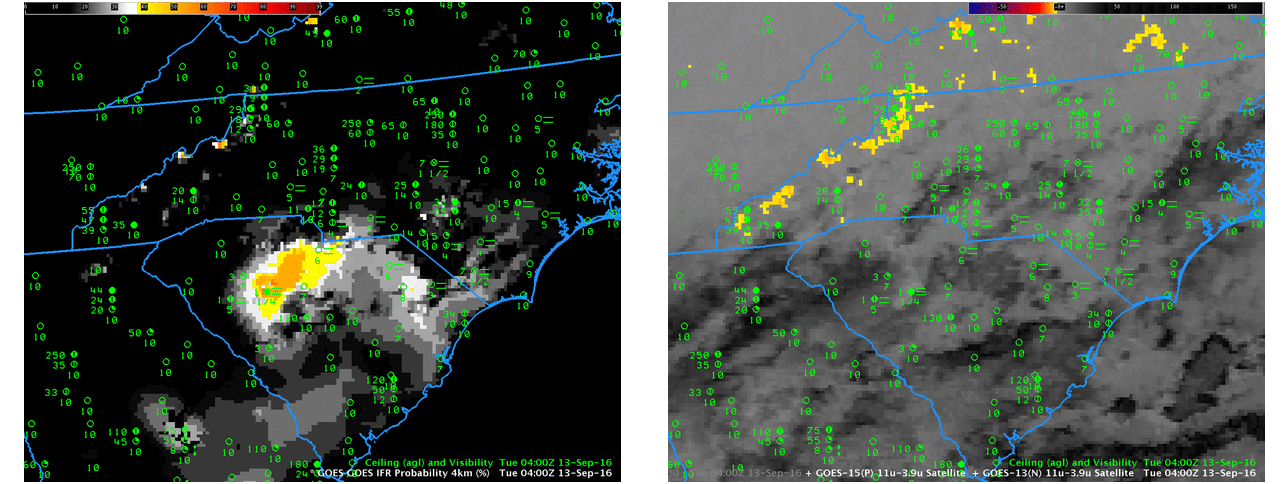
GOES-R IFR Probability fields (left) and GOES-13 Brightness Temperature Difference Fields (right), both from 0400 UTC on 13 September 2016 (Click to enlarge)
GOES-R Cloud Thickness fields can be used to estimate fog dissipation time for radiation fog. This scatterplot shows a rough relationship between the last thickness field produced before twilight conditions and the dissipation time. That field is shown below — note that portions of eastern North Carolina have slipped into twilight conditions already by 1100 UTC. Maximum values over South Carolina are around 850 feet (near Greenville/Spartanburg), while those over North Carolina exceed 1200 (near Asheville). Fog dissipation should occur first over South Carolina, then over North Carolina.